Fasting has been known to be a therapy since ancient days and Hippocrates the Father of Medicine used this to ‘cure’ epilepsy or seizures.
Fasting has also been recorded as a ‘cure’ for epilepsy in the Bible.
The ketogenic diet (KD) based on fasting’s therapeutic principle was introduced in the early 1920s. It was however introduced in India and Asia as late as 1996 by Dr J Nathan and his team. The scope of the KD has expanded well beyond epilepsy to include autism, the cancers, and neurodegenerative disorders like dementias (Alzheimer’s being the most common), ataxias, Parkinson’s disease and Parkinsonism and many more. The ‘keto’ diet has recently become a fad diet for weight loss and wellness. However, the KD, being fairly rigid, is not easy to maintain for prolonged periods. Many of the benefits of the KD like ketogenesis, promotion of potent changes in metabolic pathways and cellular processes such as stress resistance, lipolysis and autophagy, can be achieved by intermittent fasting.
Intermittent fasting (IF)
Fasting has been known to energize and extend the lifespan and more importantly the health span.
Intermittent fasting is basically a metabolic switch between glucose and ketone utilization resulting in enhancement of brain and body functionality and increasing stress, injury and disease resistance. Thus, repeating this switch between fasting and recovery (feeding) phase can lead to a healthier lifestyle. IF repairs DNA damage, enhances mitochondrial health, improves autophagy, and lowers insulin-like growth factor (IGF-1) amongst other effects. Some of the other effects are a reduction in fat mass without loss of non-fat mass (muscle), lowered glucose and insulin levels and reduction in insulin resistance. Let us explain these in simpler terms. A key study showed that IF 14 h daily for 30 consecutive days was associated with an anticancer serum proteomic signature and also upregulated the key regulatory proteins of glucose and lipid metabolism, insulin signaling, circadian clock, DNA repair, cytoskeleton remodeling, immune system and cognitive function, and resulted in a serum proteome protective against cancer, metabolic syndrome, inflammation, Alzheimer’s disease, and several neuropsychiatric disorders. Importantly, these findings occurred in the absence of any calorie restriction and significant weight loss.
Intermittent fasting is basically of three types
- Alternate day fasting – periodic fasting (PF)
- 5:2 diet: 5 days normal intake with 2 days low calorie intake (500 calories) PF
- 16:8 fasting – 16 hours fasting with 8 hours feeding or time-restricted feeding (TRF)
We recommend the last method – TRF
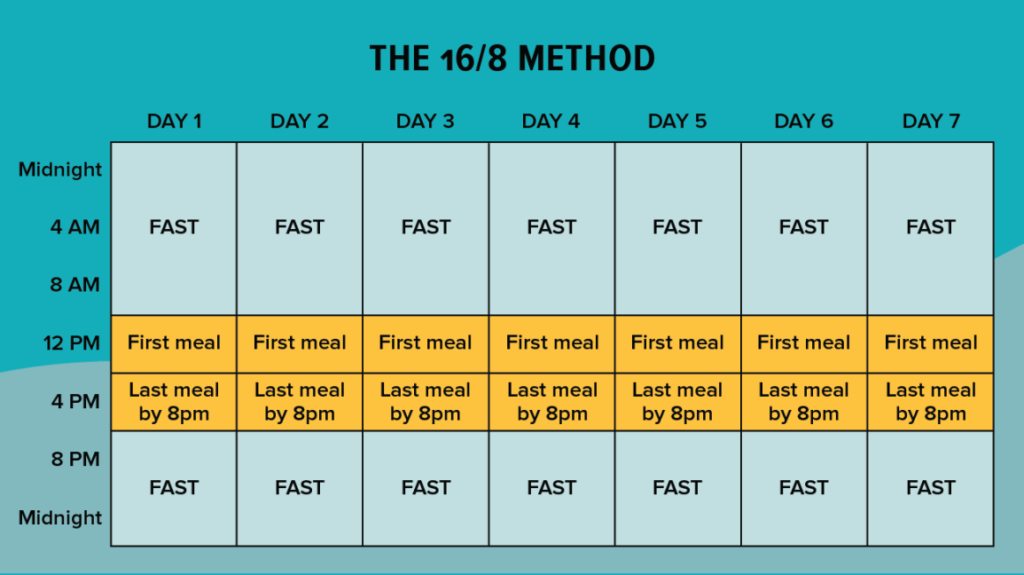
In all these modes there is an intermittent metabolic switch (IMS) which relates to the switch between glucose being used as the primary fuel during the fed state and ketones being used in the fasting state. This is called the glucose to ketone switch during the fasting state (G-K) and the reverse namely ketone to glucose switch in the fed state (K-G). Ketones gives 27% more energy as compared to glucose and in fact is the preferred fuel for the brain. More about this below.
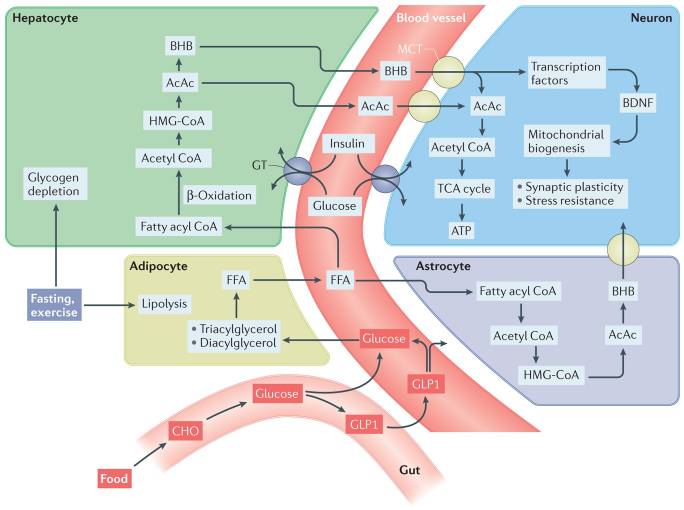
Biochemical pathways involved in the metabolic switch
During fasting and sustained exercise, liver glycogen stores are depleted and lipolysis of triacylglycerols and diacylglycerols in adipocytes generates free fatty acids (FFAs), which are then released into the blood. The FFAs are transported into hepatocytes, where they are metabolized via β-oxidation to acetyl CoA, which is then used to generate ketones – acetone, acetoacetate (AcAc) and β-hydroxybutyrate (BHB). BHB and AcAc are transported from the blood into the brain and then into neurons via monocarboxylic acid transporters (MCTs) in the membranes of vascular endothelial cells and neurons. Within neurons, BHB and AcAc are metabolized to acetyl CoA, which then enters the tricarboxylic acid (TCA) cycle (energy producing mechanism) in mitochondria, resulting in the production of ATP (the basic energy fuel) and the reducing agents that transfer electrons to the electron transport chain (energy producers). BHB can also upregulate the expression of brain-derived neurotrophic factor (BDNF) and may thereby promote mitochondrial biogenesis, synaptic plasticity and cellular stress resistance. In addition to blood-borne ketones, astrocytes are capable of ketogenesis, which may provide an important local source of BHB for neurons. Intermittent metabolic switching also increases insulin sensitivity, thereby enhancing uptake and utilization of glucose by neurons. Upon refeeding, ingested carbohydrates (CHO) and glucose stimulate release into the blood of the incretin hormone glucagon-like peptide 1 (GLP1) from enteroendocrine cells in the gut. GLP1 enhances clearance of glucose from the blood by stimulating insulin release from the pancreas and increases the insulin sensitivity of cells. GLP1 crosses the blood–brain barrier and can act directly on neurons to promote synaptic plasticity, enhance cognition and bolster cellular stress resistance. HMG-CoA, 3-hydroxy-3-methylglutaryl-CoA; GT, glucose transporter.
Upon refeeding
Mark Mattson and colleagues at the National Institute on Aging pioneers in the fasting research claim: “Upon refeeding, ingested carbohydrates* and glucose stimulate release into the blood of the incretin hormone glucagon-like peptide 1 (GLP1) from enteroendocrine cells in the gut. GLP1 enhances clearance of glucose from the blood by stimulating insulin release from the pancreas and increases the insulin sensitivity of cells. GLP1 crosses the blood–brain barrier and can act directly on neurons to promote synaptic plasticity, enhance cognition and bolster cellular stress resistance.”
Thus, this metabolic switch has potent actions on the entire body and brain leading to a happier, healthier and longer life.
In brief, IF leads to
- Reduced inflammation and reactive oxygen species (ROS)
- Increased insulin sensitivity and lowered insulin levels
- Effect on Insulin-growth factor-1(IGF-1) – a growth hormone and HGH (human growth hormone)
- Repair DNA damage and activate repair genes and pathways
- Increased autophagy – cellular repair
- Increased stress resistance
- Increased mitochondrial biogenesis
- Increased neurogenesis and synaptic plasticity
- Improved circadian rhythmicity
- Modulation of the gut microbiota
Inflammation:
Inflammation is a biological response of the immune system that can be triggered by a variety of factors, including pathogens, damaged cells and toxic compounds or irradiation. Inflammation is the immune system’s response to the above harmful stimuli and acts by removing injurious stimuli and initiating the healing process. Inflammation is therefore a defense mechanism that is vital to health. These factors may induce acute and/or chronic inflammatory responses in the heart, pancreas, liver, kidney, lung, brain, intestinal tract and reproductive system, potentially leading to tissue damage or disease. Both infectious and non-infectious agents and cell damage activate inflammatory cells and trigger inflammatory signaling pathways, most commonly the NF-κB, MAPK, and JAK-STAT pathways.
Chronic inflammation can start as an acute inflammation, example an ulcer (acute inflammation) induced by chewing tobacco which over time can turn cancerous (chronic inflammation) or a gastric ulcer (acute inflammation) turning into a stomach cancer (chronic inflammation). The etiologies of inflammation as mentioned earlier can be infectious or non-infectious. Therefore, uncontrolled acute inflammation can become chronic and lead to several diseases affecting almost all organs and can also lead to the cancers.
Inflammation triggers a leukocyte response (WBC) resulting in a high WBC count as seen in any acute inflammation. This in turn causes release of cytokines (inflammatory molecules) which induce the inflammatory response.
This response regardless of the organ triggers a common mechanism consisting of the following:
1) cell surface pattern receptors recognize detrimental stimuli;
2) inflammatory pathways are activated;
3) inflammatory markers are released; and
4) inflammatory cells are recruited.
In summary, inflammation is frequently a key element in the pathological progression of organ disease. Three main pathways, NF-κB, MAPK, and JAK-STAT, play major roles in inflammation and dysregulation of one or more of these pathways may lead to inflammation-associated disease.
IF acts positively on various pathways involved in the inflammation cascade.
IF and insulin resistance (IR)
Insulin resistance means that the cells become insensitive to insulin action. Normally, insulin pushes cells to utilize glucose thus keeping glucose levels normal. However, in insulin resistance this does not occur, and glucose levels rise resulting in higher insulin secretion by the pancreas causing higher blood insulin levels (hyperinsulinemia). At some stage the pancreas is unable to keep pace with this and IR develops. This is at the heart of most chronic disorders for example type 2 diabetes, heart attacks, paralytic attacks, dementias (Alzheimer’s), cancers etc.
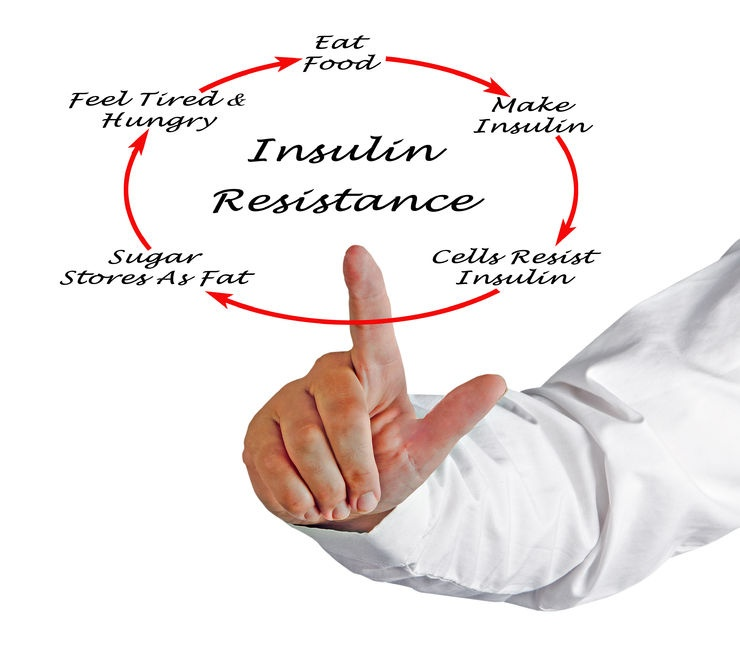
So, what are the signs you may be developing IR?
IR takes several years to develop and comes on silently.
Some pointers:
- Large waist size
- Dark patches on elbows, knuckles and armpits
- High triglycerides and low HDL (high-density lipoprotein)
- High blood pressure, high blood sugar and high fasting blood sugar
Why does insulin resistance develop?
While genetics, aging and ethnicity play minor roles in developing IR, the driving forces behind insulin resistance include excess body weight, too much belly fat, a lack of exercise, smoking, and even cheating on sleep. So do not blame your genes or even age for IR
IF reduces insulin resistance and may be better than calorie restriction, A recent study showed that there was a 52% reduction in fasting insulin at 12 months among alternate-day fasters vs. a 14% reduction among the calorie restrictors (P < .05). At the same time point, insulin resistance declined by 53% for those fasting on alternate days and by 17% for those restricting calories (P < .05). Time-based IF (TRF) is a good alternative to alternate day fasting.
IF and IGF-1
IF lowers insulin-like growth factor (IGF-1), which has strong relationships with cancer risk, aging, diabetes and many other non-communicable diseases. High IGF-1 levels are associated with aging and certain cancers. In direct contrast, those individuals with hereditary IGF-1 deficiencies (low IGF-1) appear to enjoy vast protection from age-related cancers and diabetes. IF lowers IGF-1 levels even more than calorie restriction.
IF and human growth hormone (HGH)
HGH has powerful anti-aging and longevity benefits when produced naturally in your body. More specifically, HGH can improve cognition and improve neuroprotection, helping preserve brain health.
Intermittent fasting has been shown to naturally increase HGH levels to provide anti-aging and longevity benefits for brain health.
Repair DNA damage
Sirtuins are the main DNA repair agency and are activated by IF
IF and autophagy
Autophagy is an essential house-keeping exercise is best performed during sleep (See previous chapter on Sleep). Simply put this is the mechanism by which damaged and broken cell parts are recycled. Autophagy literally means eating one’s self
Mechanistic target of rapamycin (mTOR; also known as serine/threonine-protein kinase mTOR (MTOR) and mammalian target of rapamycin). In the fed state, activation of mTOR by upstream nutrient-sensing proteins activates ribosomal protein S6 kinase increase general protein synthesis and activates sterol regulatory element-binding protein (SREBP1; also known as SREBF1) to increase lipid synthesis. Conversely, during fasting and extended exercise, when the metabolic switch is on, mTOR activity decreases. In essence cells are in a ‘growth mode’ when mTOR is active and in a ‘conserve-resources mode’ when the mTOR pathway is inactive. During fasting and sustained exercise, the mTOR pathway is inhibited and autophagy is stimulated in a wide range of tissues, including those in the nervous system. The metabolic shift to ketogenesis is not only associated with the conserve-resources mode of cells but may also be causally involved because ketones (BHB) can itself stimulate autophagy. Indeed, fasting or treatment of animals with the mTOR inhibitor rapamycin can extend lifespan and health span in several different organisms, including mice
IF and effects on mitochondria
Mitochondria are the power houses or batteries of every cell. They also control growth through mitochondrial DNA (mtDNA)
IF promotes mitochondrial biogenesis, that is increased manufacture of new mitochondria while removing damaged ones. IF also promotes homeostasis and plasticity of mitochondria thus maintaining a ‘youthful’ state. Further, it enhances mitochondrial fatty acid oxidation thus metabolizing ketone bodies. IF leads to an increase of adiponectin that interacts with adenosine 5′-monophosphate-activated protein kinase (AMPK) and stimulates Peroxisome proliferator-activated receptor gamma coactivator 1-alpha (PGC-1α) protein expression and mitochondrial biogenesis.
However the functions of mitochondria are multiple as shown below.
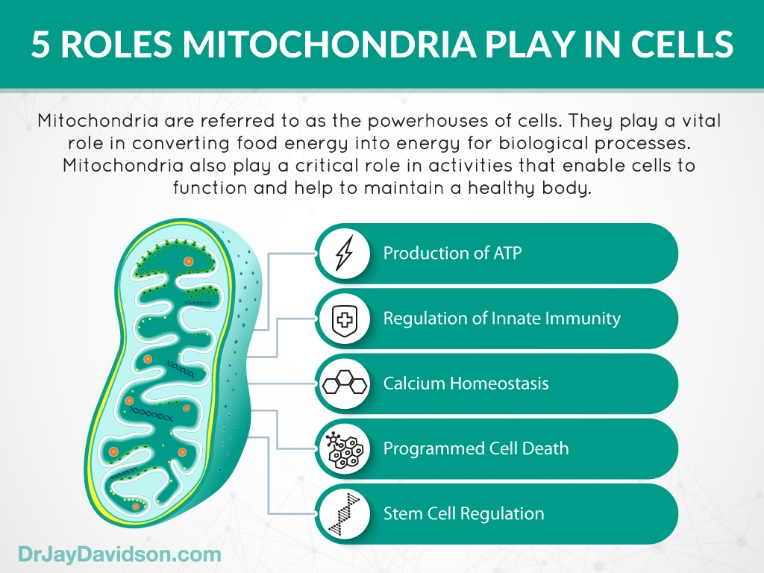
IF and neurogenesis and synaptic plasticity
Neurons are the main cells in the brain that are responsible for all the communications within the brain and to the body. There are over 100 billion neurons and they communicate through synapses. There are about 3 trillion synapses. Synaptic plasticity is the ability of the neurons to communicate with each other. They are like speakers some shout while others whisper. This organization, reorganization and rewiring of synapses is responsible for all learning.
IF increases brain-derived neurotrophic factor (BDNF) (by up to 400%) and CREB. This same mechanism is seen with ketones (from KD), exercise and high temperatures. While all three IF regimens showed an increase in proteins that regulate hippocampal neurogenesis, only IF16/8 caused a significant increase in most of these protein expressions.
Thus, IF increases new neuron formation or neurogenesis. Also, it enhances synaptic plasticity an essential requirement for memory and new learning.
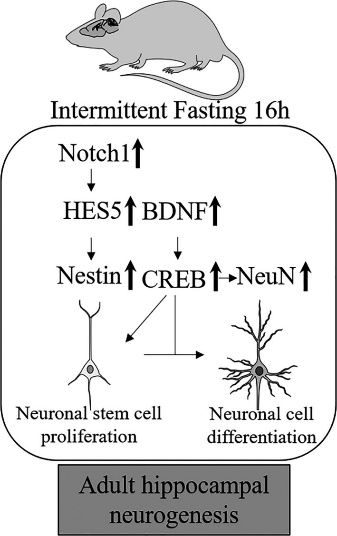
IF and gut microbiota
Hippocrates said “death sits in the bowels” and “bad digestion is the root of all evil” in 400 B.C. So, the pathogen effect was the dominant theme of the relationship between humans and our microbiomes resident in our GI tract. However, since several years we are realizing the positive role of commensal organism in our ‘healthy’ lives and the role of the ‘gut-brain’ axis – a 2-way communication system.
It is estimated that the human microbiota contains as many as 1014 types of bacterial cells, a number that is 10 times greater than the number of human cell types present in our bodies. The microbiota colonizes virtually every surface of the human body that is exposed to the external environment. Microbes flourish on our skin and in the genitourinary, gastrointestinal, and respiratory tracts. By far the most heavily colonized organ is the gastrointestinal tract (GIT); the colon alone is estimated to contain over 70% of all the microbes in the human body. The human gut has an estimated surface area of a tennis court (200 m2) and, as such a large organ, represents a major surface for microbial colonization. We have over tens of trillions of microbes in our gut, including at least 1000 different species of known bacteria with more than 3 million genes (150 times more than human genes). Microbiota can, in total, weigh up to 2 kg. One-third of our gut microbiota is common to most people, while two-thirds are specific to each one of us. In other words, the microbiota in your intestine is like an individual identity.
Additionally, the GIT is rich in molecules that can be used as nutrients by microbes, making it a preferred site for colonization. The human gut microbiota is dominated by only 2 of the 50 odd phyla resident, namely the Bacteroidetes and the Firmicutes. Colonization of the human gut with microbes begins immediately at birth. Upon passage through the birth canal, infants are exposed to a complex microbial population. Evidence that the immediate contact with microbes during birth can affect the development of the intestinal microbiota comes from the fact that the intestinal microbiota of infants and the vaginal microbiota of their mothers show similarities. Additionally, infants delivered through cesarean section have different microbial compositions compared with vaginally delivered infants.
Cyclical changes in the gut microbiome resulting from diurnal feeding and fasting rhythms contribute to the diversity of gut microflora and represent a mechanism by which the gut microbiome affects host metabolism.
Why are they important?
Because they affect various aspects on health and disease in us.
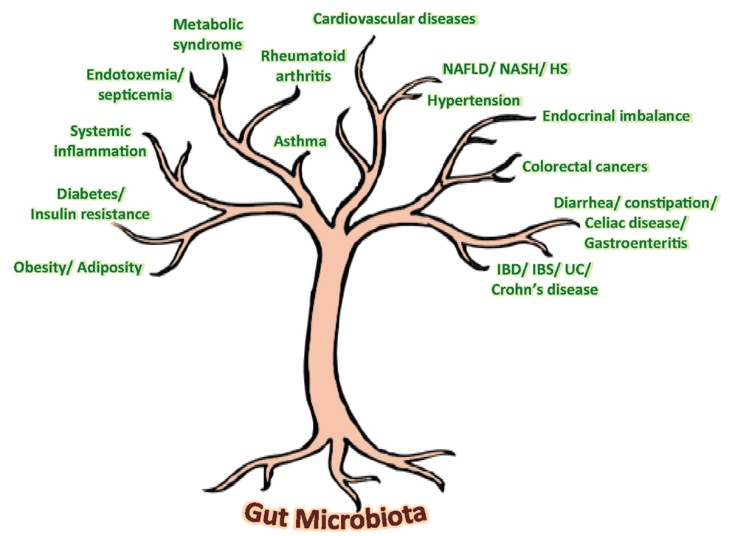
NAFLD, nonalcoholic fatty liverdi sease; HS, hepatic steatosis; NASH, nonalcoholic steatohepatitis. This can lead to hepatic cancer. |
So gut microbiota dysbiosis plays a major role in host (human) disease. Good balance of gut microbiota plays a major role in host immunomodulation, protection of the host from external pathogens, GI tract maturation, peristalsis, and barrier fortification (remember the ‘leaky’ gut syndrome). It also has effects outside the GI tract, namely, cardiac function, CNS development, appetite control and setting of the hypothalmiac-pituitary-adrenal (HPA) axis that controls our response to stress.
With such a wide spectrum of actions the influence of IF on gut microbiota is of great importance.
An extended fasting period (i.e., gut rest) could also lead to reduced gut permeability (leaky gut syndrome) and, as a result, to blunted postprandial endotoxemia and to blunted systemic inflammation, which are typically elevated in obesity. (Read our page on Leaky gut syndrome and its therapy.) Recently, investigators from the Salk Institute for Biological Studies reported that a brain–gut pathway activated in the brain during fasting acts to promote energy balance by enhancing gut epithelial integrity. Finally, jet-lag-induced dysbiosis in both mice and humans promotes glucose intolerance and obesity.
IF in humans can increase the ‘good’ gut bacteria; example Akkermansia muciniphila and Bacteriodes fragilis. Studies in rodents have shown that IF increased the levels of Firmicutes and reduced the levels of Bacteroidetes and Verrucomicrobia. These changes in the gut microbiota was associated with prolongation of survival.
Li G et al showed that intermittent fasting promotes white adipose browning and decreases obesity by changing the gut microbiota. The altered functions of gut microbiota in fasting group induce beige formation (white adipose tissue to brown adipose tissue transition-which is a beneficial reaction). Brown fat uses more thermic energy thus improving general health and weight loss. Gut microbiota increase fermentation products such as acetate and lactate during fasting. These products together with upregulation of monocarboxylate transporter 1 expression in beige cells, ameliorates obesity, insulin resistance and hepatic steatosis
Fasting switches on a gene in the gut which has anti-inflammatory effects and protects the body and the microbiome. IF has also shown to be effective in the ameliorating the clinical course of autoimmune diseases of the central nervous system such as multiple sclerosis. These effects are thought to be partially due to enrichment of the anti-inflammatory microbiota by IF.
When should the fasting state be during the day or night?
Circadian rhythm
We have evolved to be in synchrony with the day/night cycle, i.e., a circadian rhythm. Our metabolism has adapted to daytime food, nighttime sleep. Nighttime eating is associated with a higher risk of obesity, as well as diabetes. IF maintains this circadian rhythm in synchronization with the day night schedule.
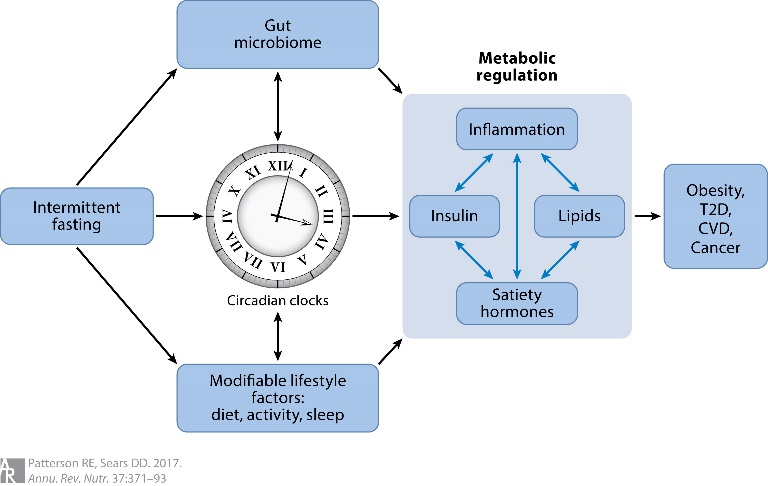
Circadian rhythms occur across 24-hour light–dark clock cycles and include changes in biology and behavior. Desynchronization of the suprachiasmatic nucleus master clock in the brain and peripheral circadian clocks in liver, fat, and skeletal muscle cells may increase the risk of chronic diseases. Feeding signals appear to be the dominant timing cue for the rhythms of peripheral clocks, including those that control metabolic pathways. Thus, consuming energy outside the normal feeding phase (i.e., late-night eating in humans) may reset some peripheral clocks and disrupt energy balance
As circadian rhythm synchronizers, it is hypothesized that fasting and time-restricted feeding regimens that actively impose a diurnal rhythm of food intake aligned with the 24-hour light–dark cycle lead to improved oscillations in circadian clock gene expression, the reprogramming of molecular mechanisms of energy metabolism, and improved body weight regulation. Thus, fasting during the night and morning hours may be more synchronous with our circadian rhythm than daytime fasting.
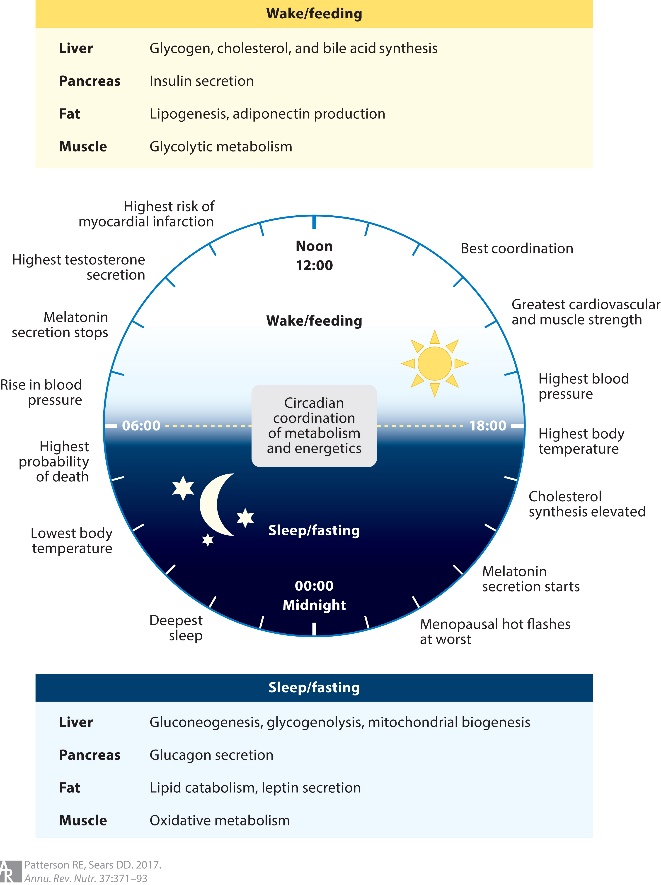
Summary of molecular changes in Intermittent Metabolic Switching (IMS)
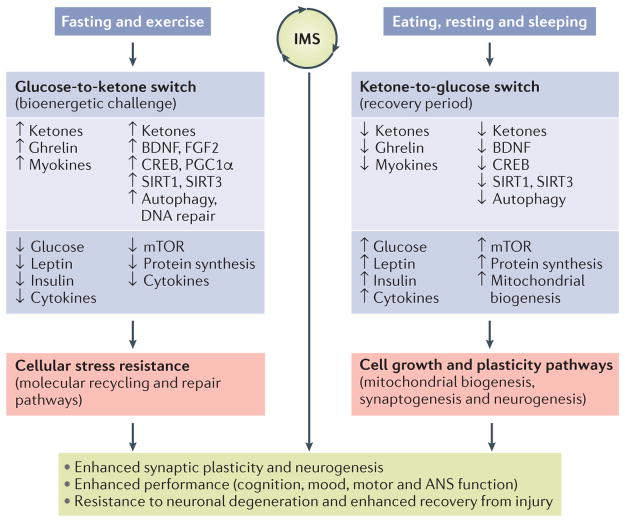
Model for how intermittent metabolic switching may optimize brain performance and increase resistance to injury and disease
Intermittent metabolic switching (IMS) involves repeating time periods of a bioenergetic challenge (fasting and/or exercise) when the metabolic switch is on (that is, liver glycogen stores are depleted and ketones are produced) and recovery periods (eating, resting and sleeping) when the metabolic switch is off. When the metabolic switch is on, levels of circulating ketones, ghrelin and myokines are elevated, whereas levels of glucose, leptin, insulin and pro-inflammatory cytokines are maintained at low levels. Adaptive responses of neurons to fasting and exercise include utilization of ketones as an energy substrate; increased expression of brain-derived neurotrophic factor (BDNF) and fibroblast growth factor 2 (FGF2); activation of the transcription factors cAMP-responsive element-binding protein (CREB) and peroxisome proliferator-activated receptor γ coactivator 1α (PGC1α), which induce the expression of genes involved in synaptic plasticity, neurogenesis and mitochondrial biogenesis; and upregulation of autophagy and DNA repair pathways. During the bioenergetic challenge, activity of the mammalian target of rapamycin (mTOR; also known as serine/threonine-protein kinase mTOR (MTOR) and mechanistic target of rapamycin) pathway and global protein synthesis are reduced in neurons, and levels of pro-inflammatory cytokines in the brain are reduced. Collectively, the pathways activated when the metabolic switch is on enhance neuronal stress resistance and bolster repair and recycling of damaged molecules. When the metabolic switch is off, circulating levels of glucose, leptin, insulin and pro-inflammatory cytokines increase, whereas levels of ketones, ghrelin and myokines decrease. In brain cells, the mTOR pathway is active, protein synthesis increases and mitochondrial biogenesis occurs during the recovery period. The activation state of neurotrophic signaling and adaptive cellular stress-response pathways may decrease when the metabolic switch is off. Cycles of IMS may optimize brain health by promoting synaptic plasticity and neurogenesis, improving cognition, mood, motor performance and autonomic nervous system (ANS) function and bolstering resistance of neurons to injury and neurodegenerative disease. SIRT, NAD-dependent protein deacetylase sirtuin. Sirtuins (1-7) are signaling proteins that are implicated in influencing cellular processes like aging, transcription, apoptosis and inflammation.
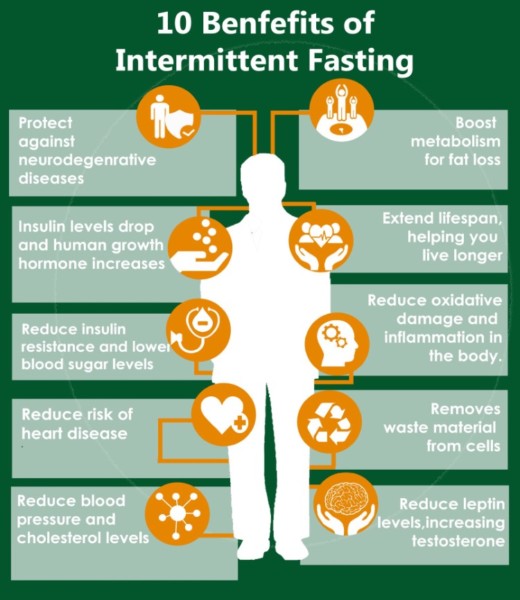
The spectrum of IF action on health and disease
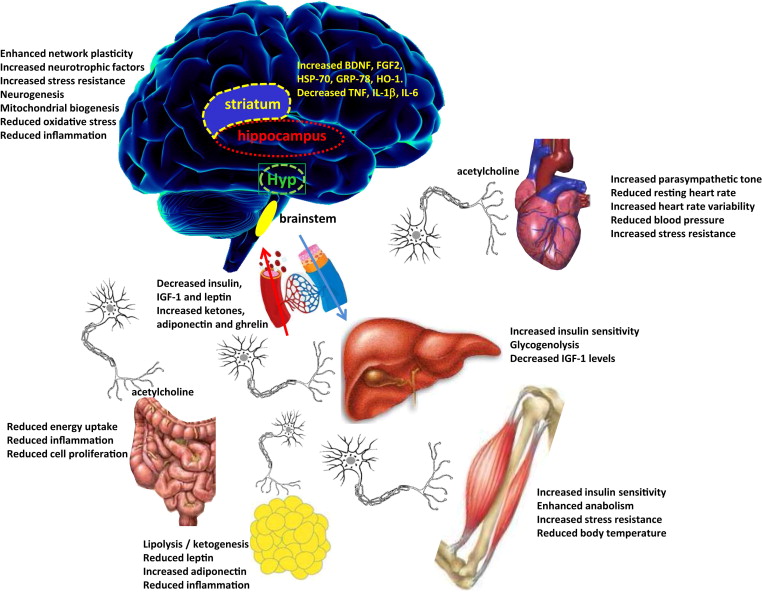
IF and disease states
IF and obesity
Carbohydrates are converted to glucose. Excess carbohydrates, especially simple carbohydrates like sugar refined wheat, polished rice are stored in the body as fat (lipogenesis). Glucose increases insulin levels which in turn promotes lipogenesis (fat storage) and inhibits lipolysis (fat removal). IF lowers glucose levels and consequently insulin levels promoting lipolysis thus resulting in weight loss.
IF and atherosclerosis, hypertension and cardiac health
IF reduces LDL (the ‘bad’) cholesterol and increases HDL (the ‘good’) cholesterol thus reducing the risk of atherosclerosis. Also, IF decreases the blood pressure and heart rate by reducing the activity of the sympathetic nervous system. The blood pressure and heart rate are also affected by the decreased production of catecholamines (norepinephrine and epinephrine) as IF decrease the level of dopamine beta-hydroxylase (the enzyme required for the production of catecholamines). In a recent study IF has shown to be effective against cardiac hypertrophy. Moreover, the oxidative stress, fibrosis and inflammation in the ageing heart are reported to be attenuated by IF.
IF and Cancers –
The potential of fasting to prevent cancer development, retard tumor growth and improve the efficacy of chemotherapy treatments is immense and is a simple, easily achievable program. We have incorporated IF into a holistic therapy for all types of cancers.
IF and Neurodegenerative disorders (NDD) like Alzheimer’s –
NDDs have at present no therapy available to either reverse or even halt the downhill course as of now. IF has potent possibilities with a special multimodal therapy being used by us.
More on these later.
Tips for successfully implementing a time-based IF
- “Start low go slow” – especially if you are not used to fasting.
- Try and have an early dinner and then nothing till next morning, that is, till next morning – around 12 hours.
- Increase the gap by half hour every week till you reach the target of 16 hours.
- If you feel hungry have green tea anywhere up to 3-4 cups. This on its own has excellent effect as it contains a catechin called EGCG (epigallocatechin-3-gallate). Catechins are natural antioxidants that help prevent cell damage and provide other benefits
- Limit your meals to three in the 8 hours of feeding time.
- You can keep your caloric intake the same unless you want to lose weight rapidly (not a very good idea).
SO WHAT ARE YOU WAITING FOR START INTERMITTENT FASTING TODAY AND LEAD A HEALTHIER LIFE